A small-molecule drug can block a broad range of flu viruses in mice
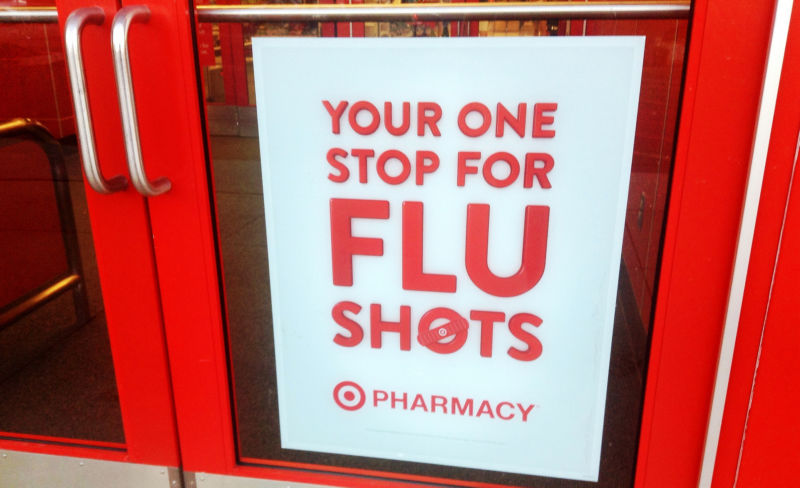
Our bodies are good at generating extremely specific antibodies, targeting a single pathogen among a dizzying mix of harmless bacteria and the proteins made by our own cells. But in some cases, like the flu virus, that specificity is limiting. Those antibodies will generally pick out a very specific strain of the flu virus, leaving us vulnerable to other strains and the new variants that evolve each season.
In recent years, however, it has become apparent that the immune system sometimes gets wildly lucky by generating a single antibody that can neutralize a huge range of viruses. These “broadly neutralizing antibodies” provide a significant protection against viruses that the immune system normally struggles against, like HIV, Ebola, and the flu virus. Mass production of these antibodies might provide a useful therapy, and the hope is that we can incorporate what they tell us into the design of future vaccines for these pathogens.
But some clever researchers have figured out how to use a broadly neutralizing antibody as a tool to design a drug that can block the activity of a large range of flu viruses.
Feeling neutral
Why doesn’t the immune system generate antibodies like this regularly? It’s a matter of evolution biting us in the rear. It’s easiest to generate an immune response to the most prominent features of a pathogen, and this generally works for clearing it out quickly. But that creates a strong evolutionary selection for mutations that change these features. As a result, the immune response is mostly an evolutionary arms race between the immune system dealing with the latest version of a virus and mutations producing even newer versions.
But on rare occasions, the immune system gets lucky and makes an antibody to a less prominent region on the pathogen. Some of those are even luckier still, and they stick to part of a protein that’s utterly essential to the pathogen and block its function. This sort of blockade is very hard to evolve around. In addition, since different strains of a virus rely on the same functions to infect a cell, these antibodies can often block all of the strains.
In the case of the flu virus, broadly neutralizing antibodies have been discovered, and these tend to bind to the virus’ hemagglutinin protein (the H in the flu’s HA nomenclature). This protein becomes important after a flu virus attaches to a cell and is brought inside. The hemagglutinin senses this change of conditions and undergoes a rearrangement that allows the virus’ genetic material to enter the cell. Broadly neutralizing antibodies blocks this process, essentially trapping the virus’ genetic material where it ends up being harmlessly digested. Typically, they’ll block all the viruses that have one of the two classes of the hemagglutinin protein—a huge range of viruses.
On their own, these antibodies could potentially provide a therapy for people who are already infected. But antibodies have issues. They need to be refrigerated before use, need to be injected (because they won’t survive the digestive tract), and can end up having the immune system react to them. So, some people have tried to simplify the antibodies down, creating much smaller proteins that have a similar structure to the antibody and can also block hemagglutinin. And that’s where the new research comes into the picture—literally and figuratively displacing the antibody.
Displacing an antibody
The researchers—an enormous partnership among academic labs and Johnson & Johnson—figured out an extremely clever test for drug activity. They started with hemagglutinin and stuck the simplified, small version of the antibody to it. Then the researchers threw a library of about a half-million small molecules at the hemagglutinin, one at a time, and they searched for the small molecules that would cause the aforementioned simplified, small version of the antibody to fall off the hemagglutinin. This should only happen if the small molecule binds even better and thus has a good chance of blocking the virus even more effectively.
About 9,000 molecules came through the screen looking promising, and the researchers looked through them to find common features. Many of them had a specific structure (they had a benzylpiperazine group), so the researchers focused on one of those. Unfortunately, this chemical doesn’t dissolve in water very well, and tests in mice showed that it was cleared from their system rapidly. So, the researchers made some changes that added more oxygen to the structure to make it more soluble. A few additional changes increased the strength of the binding by roughly a factor of 50.
To test whether it works, the authors turned to an extreme and somewhat contrived situation: they started giving the mice the drug. A day later, the researchers hit the mice with a lot of the flu virus—25 times the dose that would normally kill half of them. With the drug, however, all of the rodents lived.
Humans
Obviously, ethical considerations prevent us from doing a similar experiment in humans. So the authors developed a three-dimensional culture of human bronchial cells and infected it with the virus. Again, the drug effectively blocked infection.
There’s a lot more to the research, including an exploration of what it would take to have a drug that blocked the second major class of hemagglutinin. But really, the important work is going to be in experiments that aren’t reported here: things like toxicity and side effects, ability to get into the bloodstream when in pill form, and whether the drug can block consequences of infections after they’ve started. These things will determine whether pursuing this (or any other related chemicals) as potential therapies is worthwhile.
In either case, however, the basic idea here—using broadly neutralizing antibodies as tools to identify virus blocking drugs—may work for many other viruses. So, even if this chemical doesn’t work out, the potential is there to identify other troublemakers, including even deadlier viruses.
Science, 2019. DOI: 10.1126/science.aar6221 (About DOIs).
https://arstechnica.com/?p=1471727