Physicists captured, quantified the sound of champagne’s effervescence
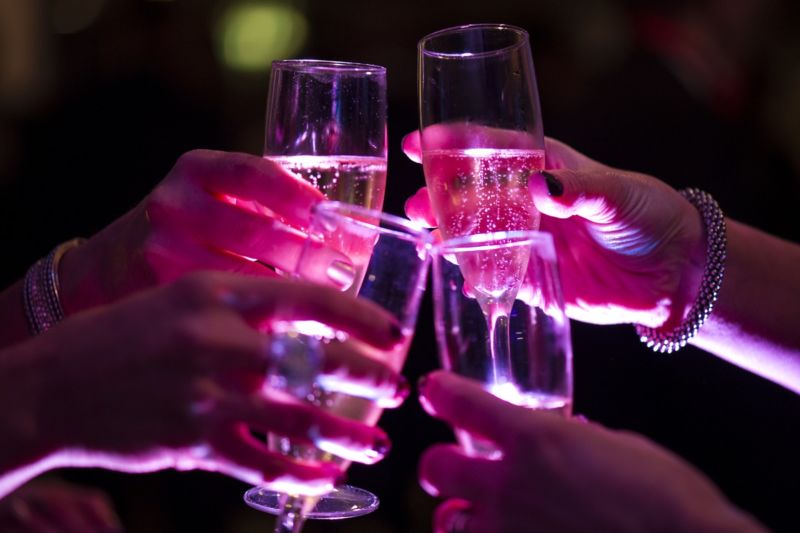
There’s nothing quite like the distinctive crackling and fizzing sound of a glass of freshly served champagne. It’s well established that the bursting of the bubbles produces that sound, but the specific physical mechanism isn’t quite clear. So physicists from Sorbonne University in Paris, France, decided to investigate the link between the fluid dynamics of the bursting bubbles and the crackly fizzy sounds. They described their work in a paper published back in January in the journal Physical Review Fluids.
As we’ve reported previously, the first mention of a sparkling wine dates back to 1535 in the Languedoc region of France. The classic brand Dom Perignon gets its name from a 17th-century monk who had the job of getting rid of the bubbles that developed in his abbey’s bottled wine, lest the pressure build up so much they exploded. Legend has it that upon sipping such a bubbly wine, the monk realized the bubbles might not be such a bad thing after all, declaring, “Come quickly, brothers, I am drinking stars!”
In the 18th century, British chemist Joseph Priestley invented an artificial carbonation process while living next to a brewery in Leeds. Ever the scientist, he started experimenting with the CO2 used by the brewery and found that a bowl of water placed above a fermenting liquor became slightly acidic to the taste, just like natural mineral waters. He included his simple instructions for artificial carbonation in a 1772 treatise, Impregnating Water with Fixed Air.
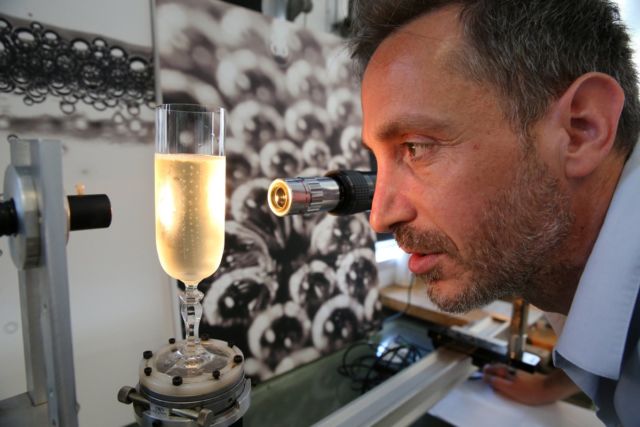
Carbonation is a particularly fascinating topic within the subfield of fluid dynamics. For instance, a 2018 article in Physics Today reported that carbonation triggers the same pain receptors in our deep brains that are activated when we eat spicy food. Other fun facts gleaned from champagne science over the years: when the bubbles in champagne burst, they produce droplets that release aromatic compounds believed to enhance the flavor further.
Also, the size of the bubbles plays a critical role in a really good glass of champagne. Larger bubbles enhance the release of aerosols into the air above the glass—bubbles on the order of 1.7mm across at the surface. And the bubbles in champagne “ring” at specific resonant frequencies, depending on their size. So it’s possible to “hear” the size distribution of bubbles as they rise to the surface in a glass of champagne.
The latter is the only study to date specifically examining the acoustic emissions (crackling and fizzing) of champagne specifically, according to the authors of this latest paper. But there were two prior studies in 1992 and 2013 focusing on the the acoustic emission of bubbles collapsing at a water surface more generally, revealing that the smallest bubbles emitted more of a chirp.
Champagne’s effervescence arises from the nucleation of bubbles on the walls of the glass. Once they detach from their nucleation sites, the bubbles start to grow as they rise to the liquid surface, bursting and collapsing at the surface. This typically occurs within a couple of milliseconds, and the distinctive crackling sound is emitted when the bubbles rupture.
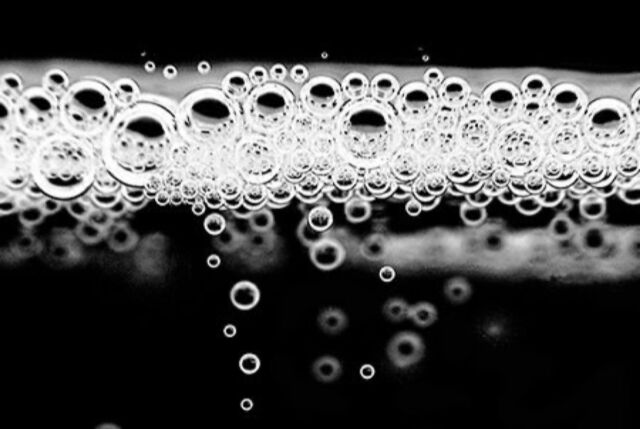
The French physicists used a glass tank containing tap water, and a tank containing of a water/surfactant solution for their experiments, since champagne also contains a small volume of surfactant molecules. They injected air bubbles into the tanks using submerged needles connected to a syringe pump filled with air. The bubbles would rise to the surface and float briefly before bursting. All of this was captured on video with two digital high-speed cameras, while the acoustic emissions (sounds) were recorded by a microphone positioned just above the liquid surface. Finally, they filtered the acoustic data to remove any ambient noise.
As Katherine Wright wrote at APS Physics:
Analyzing the data, Pierre and colleagues find—as expected—that the production of the sound coincides with the rupture of the bubble. As the bubble nears the surface, the pressure of the gas inside it increases. This pressure is violently released when the bubble bursts.
The bubble, however, doesn’t immediately disappear. The part of the bubble that is still submerged generates acoustic vibrations of the liquid-gas interface. The frequency of this vibration depends on the volume of gas the bubble contains and on the diameter of the hole in the bubble. As a result, the frequency changes as the rupture grows and the bubble shrinks, increasing in pitch until the bubble dies. For the small micrometer-sized champagne bubbles, only the beginning of the rupture is audible to humans, while for larger millimeter-sized bubbles, the whole burst can be heard.
This process is markedly different from how bubbles beneath the surface emit sound, and the team thinks looking for acoustic signatures could shed light on other hydrodynamic phenomena that elude conventional imaging techniques. “We believe that [our] quantitative description could be used to synthesize artificial acoustic signals of digital animation films,” the authors wrote. “More generally, this work is a step in understanding the acoustic signature of violent hydrodynamic events, which adds to previous studies on volcano eruptions… breaking waves, and bursting soap bubbles.”
DOI: Physical Review Fluids, 2021. 10.1103/PhysRevFluids.6.013604 (About DOIs).
https://arstechnica.com/?p=1822847