Physics not “broken” after all? We’re close to resolving proton radius puzzle
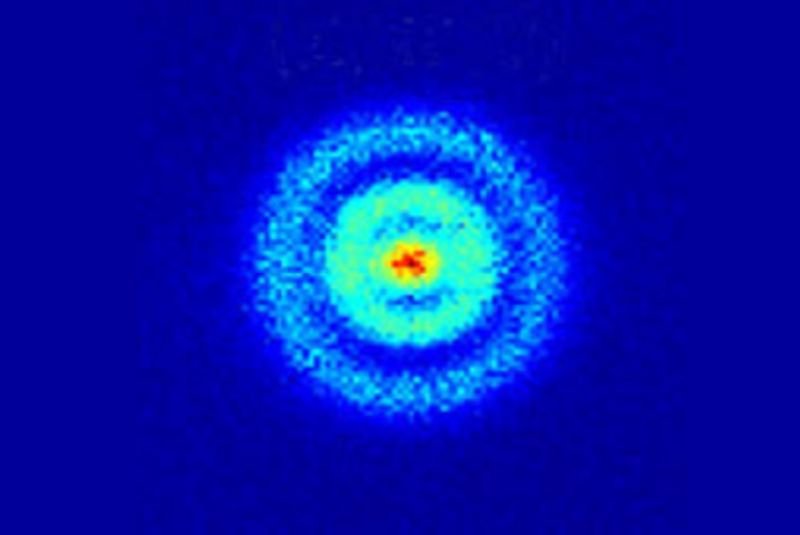
Physicists at York University in Toronto have spent the last eight years meticulously conducting a sensitive experiment to measure the charge radius of the proton in hopes of resolving conflicting values obtained by several similar experiments performed over the last decade. That conundrum has been dubbed the “proton radius puzzle.” The new results, published in a new paper in Science, confirm a 2010 finding that the proton is significantly smaller than scientists previously believed.
Most popularizations discussing the structure of the atom rely on the much-maligned Bohr model, in which electrons move around the nucleus in circular orbits. It’s fine as a gateway drug to physics, so to speak, but quantum mechanics gives us a much more precise (albeit weirder) description. The electrons aren’t really orbiting the nucleus; they are technically waves that take on particle-like properties when we do an experiment to determine their position. While orbiting an atom, they exist in a superposition of states, both particle and wave, with a wave function encompassing all the probabilities of its position at once. A measurement will collapse the wave function, giving us the electron’s position. Make a series of such measurements and plot the various positions that result, and it will yield something akin to a fuzzy orbit-like pattern.
Quantum weirdness extends to the proton, too. Technically it’s made of three charged quarks bound together by the strong nuclear force. But it’s fuzzy, like a cloud. And how can we talk about the radius of a cloud? Physicists rely on the charge density to do so, akin to the density of water molecules in a cloud. The radius of the proton is the distance at which the charge density drops below a certain energy threshold. And it’s possible to measure that radius by studying how the electron interacts with the proton, via either electron scattering experiments or by using electron or muon spectroscopy to look at the difference between atomic energy levels. (It’s called the “Lamb shift,” after Nobel laureate Wallis Lamb, who first measured the shift in 1947.) The combined fuzziness of the electron and proton means that the electron can be anywhere inside that region—including inside the proton.
Hydrogen atoms are the simplest nuclei, with a single proton orbited by an electron, so that’s typically what physicists have used for their experiments to measure the proton’s charge radius. For a long time, the accepted value was .876 femtometers—a “world average” of many different measurements with sufficient error bars to allow for future measurements.
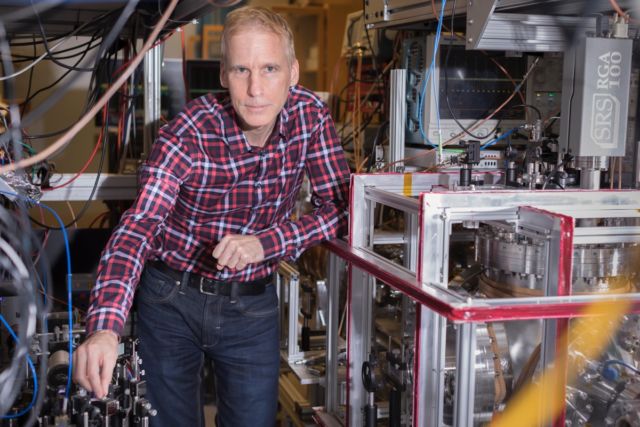
It’s the muon spectroscopy measurements that first caused the problem back in 2010. For their experiment, physicists at the Max Planck Institute of Quantum Optics used muonic hydrogen, replacing the electron orbiting the nucleus with a muon, the electron’s heavier (and very short-lived) sibling. Since it’s nearly 200 times heavier than the electron, it has a much smaller orbital, and thus a much higher probability (10 million times) of being inside the proton. And that makes it ten million times more sensitive as a measurement technique, because of its closer proximity to the proton.
Randolf Pohl, one of the physicists who performed the original 2010 experiment, explained the underlying concept at a 2013 meeting of the American Physical Society. “Assume the proton is a ball of charge, with the electron dancing through the proton,” he said. “When it is in the center of the proton, it is attracted equally from all sides, with the charges all around it, so there is no net attraction between proton and muon. This shifts the whole energy state up. That is the effect we are looking at with laser spectroscopy, when we measure the difference between two energy levels: when the electron is inside and outside the proton.”
The physicists expected to measure roughly the same radius for the proton as prior experiments, only with less uncertainty. There should be no difference (other than mass and lifetime) between the electron and the muon, theoretically. Instead, they measured a significantly smaller proton radius of 0.841 femtometers, 0.00000000000003 millimeters smaller, well outside the established error bars. It was a strong 5-sigma result—five standard deviations out from the value obtained by other methods.
“It certainly makes a pretty strong case for the smaller size being the correct size.”
Pohl et al. spent years checking and re-checking their data, so if it was an experimental error—or if the underlying theory of quantum electrodynamics (QED, which describes how light interacts with matter) was somehow misapplied—it’s a significant one. Theorists pondered whether QED might need to be tweaked to take into account possible slight differences in the muon’s properties. The most exciting possibility: this could be a hint of new physics beyond the Standard Model. This was always the least likely explanation, and over the ensuing decade, it has become even less likely.
Subsequent measurements by various groups have been inconclusive as to whether the larger or smaller value is correct. For instance, in 2013, the same international team performed muon-based experiments that confirmed their 2010 value, producing a measurement of 0.84 femtometers for the proton’s radius, with a discrepancy of 7 sigma. Another experimental variation in 2016 involved replacing the electron with a muon in a deuterium atom—a heavier isotope of hydrogen, with a neutron as well as a proton and electron. The idea was that the presence of a neutron would alter how electrons and muons perceive the proton’s charge. That, too, was in line with the 2010 result.
However, two experiments using regular hydrogen to measure the proton radius produced mixed results: a 2017 study by Theodor Hänsch’s group (including Pohl) also confirmed the 2010 result, while a 2018 measurement was in line with the larger value before the 2010 experiment. So the York University scientists recently opted to make an electron-based measurement of the proton radius, analogous to the original 2010 muon-based measurement, in hopes of bringing the various conflicting results closer to a consensus.

While the muon measurements required large particle accelerators to produce the muons, the York University team was able to perform a tabletop experiment—albeit using a rather large tabletop measuring about four meters (13 feet). And they deliberately made a blind measurement to ensure against any bias, finally revealing the value they had measured over eight years just a few weeks prior to submitting their paper for publication. “The difficulty is making sure we’re not influenced by anything that could complicate or shift energy states in our measurement,” said group leader Eric Hessels. “A lot of the eight years [were] spent taking great care in understanding all aspects of the measurement so that we can carefully eliminate possibilities of having made mistakes.”
The result: their measurement of 0.833 femtometers (just under one-trillionth of a meter) agrees with the smaller value from the 2010 study. That’s good news for the Standard Model and bad news for those hoping for some exciting new physics. “Because it’s a direct comparison, it certainly makes a pretty strong case for the smaller size being the correct size,” said Hessels. Additional experiments by other groups are currently underway, and he expects the community will converge on a consensus as those results trickle in over the next couple of years.
“Fundamentally, we really want to understand what all the laws of physics are, and if there’s a discrepancy that nobody can explain, there is a possibility that the laws of physics are not understood,” said Hessels of the motivation for doing such experiments. “If we do agree on a smaller size for the proton, that becomes another building block of what we do understand, and allows us to use the hydrogen atom to test other things: to test the theory of quantum electrodynamics at higher precision, or to test whether there is anything else beyond the Standard Model of physics.”
DOI: Science, 2019. 10.1126/science.aau7807 (About DOIs).
https://arstechnica.com/?p=1562299