Quantum physicists in the 1920s helped found field of quantum biology

In 1944, quantum physicist Erwin Schroedinger wrote a short book called What is Life: The Physical Aspect of the Living Cell, exploring how the relatively new field of quantum mechanics might play a role in biological processes. It is considered by many to be one of the earliest forays into “quantum biology,” a rarefied field that attempts to apply quantum principles to living systems. But the field actually dates back to the earliest days of quantum mechanics in the 1920s, according to a recent paper published in the Proceedings of the Royal Society A.
“Quantum biology is wrongly regarded as a very new scientific discipline, when it actually began before the Second World War,” said co-author Johnjoe McFadden, a microbiologist at the University of Surrey and co-director of its Centre for Quantum Biology, along with his Surrey colleague and co-author Jim Al-Khalili. “Back then, a few quantum physicists tried to understand what was special about life itself and whether quantum mechanics might shed any light on the matter.”
Frankly, quantum biology has suffered from a lack of credibility until the last decade or so, when a number of intriguing studies suggested that there might be something to the idea after all. For instance, there is growing evidence that photosynthesis relies on quantum effects to help plants turn sunlight into fuel. Migratory birds might have an internal “quantum compass” that helps them sense Earth’s magnetic fields as a means of navigation. Quantum effects might play a role in the human sense of smell, helping us distinguish between different scents.
“We’ve forgotten that there were these mavericks even before Schroedinger who were asking these deep questions.”
More controversially, mathematical physicist Roger Penrose suggested in 1989 that mysterious proteins called “microtubules” might exploit quantum effects and hold the secret to human consciousness. Few researchers believe this is actually true, but Matthew Fisher, a physicist at the University of California, Santa Barbara, has recently proposed that the nuclear spins of phosphorus atoms might function as simple “qubits” in the brain. Consciousness, in other words, would work much like a quantum computer.
That’s why McFadden and Al-Kalili wrote their bestselling popular science book, Life on the Edge: The Coming of Age of Quantum Biology in 2015. One of the chapters that didn’t make it into the final book—exploring the historical origins of the field—ended up forming the basis for this latest paper. “We’ve forgotten that there were these mavericks even before Schroedinger who were asking these deep questions that we are only now able to test a bit more carefully,” said Al-Khalili.

By 1927, physicists had laid out the mathematical framework for the new theory of quantum mechanics. “Flushed with their success at taming the atomic world, and with the arrogance of youth on their side, many quantum pioneers struck out of their physics laboratories and away from their blackboards to seek new areas of science to conquer,” the authors write. And since microbiology and the related, fledgling field of genetics remained largely unexplored, the intellectually restless physicists naturally gravitated there.
There was even a Theoretical Biology Club at Cambridge in 1932; its members included physicists, philosophers (like Karl Popper), and biologists. “They were all united in the idea that there’s something special about life,” said Al-Khalili. “They felt that maybe principles in physics and chemistry yet to be discovered could help distinguish the transition between chemistry and biology.” Granted, it was more of a hobby for many of them, and Al-Khalili concedes they didn’t make much progress. But those early discussions certainly had a great influence on Schroedinger.
Niels Bohr was not entirely convinced that the principles of physics and chemistry would be sufficient to explain living systems, but a lecture he gave at the Scandinavian Meeting of Natural Scientists in 1929 briefly mentioned the possibility. Among those inspired to delve further was a German physicist named Pascual Jordan, one of three authors of the seminal paper laying out the mathematical foundations of quantum mechanics. He was using the term “quantumbiologie” in the late 1930s and published Physics and the Secret of Organic Life—in which he explored the question of whether atomic and quantum physics is essential for life—the year before Schroedinger published What Is Life.
“They were all united in the idea that there’s something special about life.”
Unfortunately, Jordan was a devout member of the Nazi party, although his defense of Jewish scientists like Einstein meant he was deemed “politically unreliable” by that regime. His attempts to link his theories on quantum biology to the Nazi philosophy—even claiming that a single dictatorial leader (führer) was a central principle of life—served to discredit those theories in the eyes of his fellow scientists.
“Had it been other physicists, maybe people would have taken more notice [of quantum biology] and carried on thinking about some of these problems,” said Al-Khalili. “But because of Jordan’s background, it was deemed an unsavory area of research.”
So it fell to Schroedinger the keep the flame of quantum biology alive. “There’s a famous image from one of his notebooks where he drew diagrams of chromosomes, trying to understand how they’re able to store information,” said Al-Khalili. “He wanted to know what it was that kept life in this highly ordered state.” In What Is Life, Schroedinger argued that, unlike inanimate matter, living matter can be influenced by single quantum events. After all, cool certain materials down to near absolute zero and they exhibit quantum effects like superconductivity, in which the electrical resistance disappears. According to Schroedinger, living matter could also exhibit these kinds of effects at room temperatures, perhaps because it’s so highly ordered.
Specifically, he pondered how fruit flies, for instance, managed to produce order from disorder, decreasing entropy (in seeming violation of the second law of thermodynamics) by “continually sucking orderliness from the environment.” Entropy always increases in a closed system, according to physics, but living things are not isolated systems. A fruit fly might extract order from disorder, but there is a corresponding increase in entropy in its environment. Schroedinger also suggested an “aperiodic crystal” might contain genetic information and that mutations occur via “quantum leaps.”
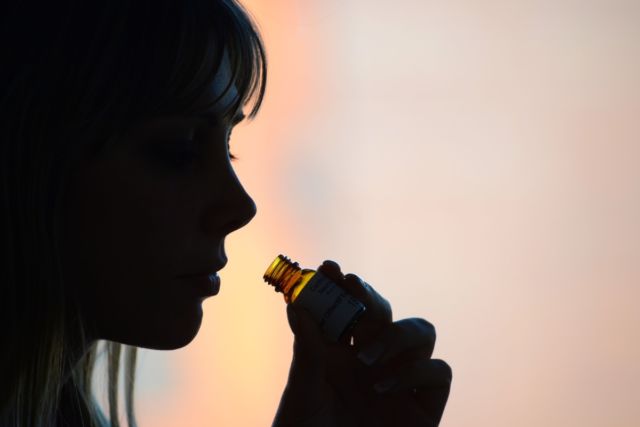
What Is Life was hugely influential at the time; Francis Crick and James Watson claimed it helped inspire them to think about the double helix structure of DNA, along with Rosalind Franklin’s X-ray diffraction experiments. But quantum biology fell out of favor after that as an area of credible research. The general consensus among physicists in the ensuing decades was that living systems were simply too noisy and quantum effects just too delicate to persist in a complicated environment like a living cell.
The issue is quantum decoherence. Entanglement is key for quantum effects: connecting two or more objects in such a way that they can only be described with reference to each other, even if separated over large distances. Albert Einstein famously dubbed it “spooky action at a distance.” But the slightest interaction (colliding with a single photon, for instance) with the surrounding environment will destroy that entanglement.
“Usually we think that the more complex the environment, the faster it decoheres, like a hot object will cool down in a cold environment,” said Al-Khalili. “If it didn’t, we would have built a quantum computer by now. So how do you maintain these delicate quantum effects long enough for them to be useful?”
Current thinking holds that there may be some living systems where quantum processes could play a role before decoherence kicks in. That’s because such systems depend on the dynamics of small numbers of molecules at tiny scales (just a few nanometers), keeping them sufficiently isolated. In fact, the authors contend that recent work in quantum information theory demonstrates that noise might actually support quantum coherence in some systems. Maybe, over billions of years of evolution, nature has learned the trick of maintaining quantum coherence to make use of such effects, and we just don’t yet understand how.
These are concepts Al-Khalili himself is only beginning to explore seriously in his research at the Centre for Quantum Biology. “For me, quantum biology is an excuse to look into the foundations of quantum mechanics; the fact that it’s inside biological systems is almost incidental,” he said. “When I first started thinking about quantum biology, it was really just a hobby. I didn’t really believe it. I can’t say I even 100 percent believe it now, but I think it’s an interesting enough problem that we have to rule it out. We’ve got to stop just waving our hands about and do some careful research.”
DOI: Proceedings of the Royal Society A, 2018. 0.1098/rspa.2018.0674 (About DOIs).
https://arstechnica.com/?p=1436107