Science 101 tells us that the twinkling appearance of stars from our vantage point on Earth is due to atmospheric effects: winds and varying temperatures and densities in the air bend and distort the light. But stars have another sort of “twinkle” produced by how gases ripple in waves across their surface, an effect that could provide astronomers with a handy means of exploring the interior of massive stars to learn more about how they form and evolve. But the effect is much too small to be readily detected by telescopes.
So scientists have now developed the first 3D simulations of that innate twinkle, according to a recent paper published in the journal Nature Astronomy. As a bonus, the researchers converted the data from those rippling waves of gas into an audible sound, so now we can all take a moment to listen to “Twinkle, Twinkle, Little Star” (see video above) and Gustav Holst’s “Jupiter” (see video below) in the “language” of the stars.
“Motions in the cores of stars launch waves like those on the ocean,” said co-author Evan Anders of Northwestern University. “When the waves arrive at the star’s surface, they make it twinkle in a way that astronomers may be able to observe. For the first time, we have developed computer models which allow us to determine how much a star should twinkle as a result of these waves. This work allows future space telescopes to probe the central regions where stars forge the elements we depend upon to live and breathe.”
The critical feature for this latest research is the so-called “convection zone,” typically found near the surface, although it can also persist deeper into the star. (Stars can also develop convection zones near the core.) Our Sun, for instance, has a convective envelope ranging from its surface to about one-third of the way toward its core. Stellar convection is what moves matter from the star’s deeper and hotter layers outward to the cooler outer layers, and material from those outer layers to the inner hotter layers.
Convection is also one proposed mechanism for the so-called “red noise” signals astronomers have observed in the photometric light curves of hot, massive stars—a mysterious pulsing that causes fluctuations in the stars’ brightness. Specifically, it’s been suggested that core convection, or turbulence from subsurface convection zones, could produce gravity waves that ripple outward to the surface. Those waves would compress and decompress the plasma, producing the brightness fluctuations in the star’s light. Anders and his colleagues developed their simulations in part to test that hypothesis. The challenge: while some waves make it to the surface, others are trapped below and bounce around. So they needed some means of distinguishing between the two kinds of waves.
To do so, they turned to an acoustic analogy. “The character of music depends both on the sound waves produced by musicians and on the acoustics of the environment where is played,” the authors wrote. “Music is recorded in special studios with walls that absorb or diffuse waves to minimize the influence of the environment on the sound and retrieve the ‘pure sound’ of the musicians. To experience music in a different environment, it is not necessary to physically transport the musicians; instead, one can apply a filter to the recording, mimicking the effects of the new environment.”
The team adopted a similar strategy, running short simulations of waves generated by convection and recording the waves as they moved beyond the convection zone. First, they built a model to calculate the basic “song” of those convection waves—technically, the photometric variability from the gravity waves—and then they applied a filter to replicate the star’s acoustical properties, akin to the damping filters used in a recording studio. Once this approach had been validated, Anders et al. ran convection simulations for stars with masses of three, 15, and 40 times our Sun. These showed what those waves should look like when viewed through a telescope.
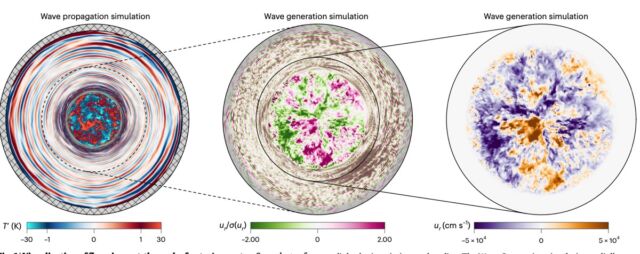
As for the sonification, a star’s convection produces waves that correspond to different sounds. “The smaller stars in our study are more like the violin, where they have some more high-pitched noises because they have a smaller wave cavity, just like a violin has a smaller wave cavity,” Anders told New Scientist. “And our larger stars have a bigger wave cavity, just like a cello has a bigger wave cavity, so they have some deeper noises.” They used their model to find out what a song would sound like if we heard to propagated through a star by applying it to real music. “The stars change the music and, correspondingly, change how the waves would look if we saw them twinkling on the star’s surface,” said Anders.
The simulations also revealed that the twinkling attributable to core convection is simply too weak to fully explain the observed red noise effect in massive stars. It’s possible that convection nearer to the star’s surface could account for the red noise, but according to co-author Matteo Cantiello of the Flatiron Institute’s Center for Computational Astrophysics in New York, this would tell astronomers less about the processes occurring deep in the star’s interior. The next step is to improve their simulations to take other effects into account, such as a star spinning rapidly around its axis, which might produce a flickering strong enough to be detected by telescopes.
DOI: Nature Astronomy, 2023. 10.1038/s41550-023-02040-7 (About DOIs).
Listing image by E.H. Anders et al., 2023
https://arstechnica.com/?p=1956539