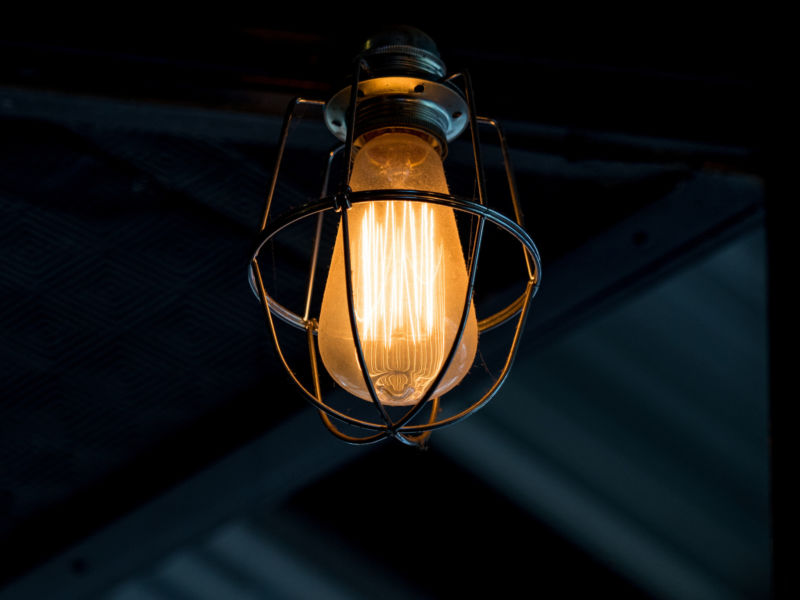
We enjoy the life of plenty when it comes to technology. We like the speed at which information flows to us, carried on the back of hard-working photons. We wax lyrical, if a bit amateurishly, about the color and clarity of OLED televisions. Without our technological mastery of light, our society would be a good deal poorer.
That mastery, though, didn’t appear out of thin air. It usually takes decades to go from the first “Wait, did that just glow?” to a technology being taken for granted.
So, what is the next big thing? No one really knows for sure, but I’m going to put in a good word for light-emitting metal-organic frameworks (MOFs). Light-emitting MOFs combine all the good things that we associate with organic light emitters with the best things about inorganic light emitters. If it all pans out, engineers will have a platform that has flexibility in design, simplicity in fabrication, and robustness in use. Before today, I wondered why I had not seen many results on light-emitting MOFs. And, now I realize that, like every other light-emitting technology, we are still in the “decade or more of work” phase with them.
Introducing empty crystals
I am not a chemist, so what follows is going to be the worst mangling of science since Uri Geller learned to talk. The basic idea behind MOFs is that we love crystals. Crystals are an ordered structure, and many of the electronic and optical properties of a material come from that structure. It would, however, be very nice to be able to control the structure, but we’re typically limited by what nature gives us. In nature, the spacing between adjacent atoms or molecules is set by the type of bond between them, and it’s never very large—think of lengths less than a nanometer.
The other problem with most crystals is that they are not very strong. Think of salt crystals with their ionic bonds: easy to break and even easier to dissolve. Likewise, if you crystallize a protein, the connections between the different protein molecules are very weak indeed. This is why crystallizing proteins is a difficult art to master. At the other end of the scale is diamond, which is anything but weak. What is the difference? Diamond is less a crystal than a single molecule, held together by covalent bonds.
If you want to get me really excited, tell me that you have a way to create strong crystals with arbitrary distances between their atoms and molecules. This is almost what a MOF is. Start with organic molecules, which are basically chains of carbon atoms. These are then attached to a single metal atom at each end. Each metal atom has room for four carbon chains. So, if you control the conditions nicely, you end up with a repeated set of cubes; the metal atoms form the corners of the cubes, while the carbon chains bind the metal atoms to each other. Our crystal spacing is given by the length of that carbon chain. It’s absolutely amazing.
MOFs, even back when I learned of them, were commercial products. They have all sorts of useful properties as molecular sponges, catalysts, filters, and many, many more. Missing from that list, though, is anything to do with light.
Light-emitting MOFs
OLEDs are basically organic molecules that emit light when a current is passed through them. OLEDs were inspired by nearly identical organic molecules that glow when you shine light on them. It did not take long for scientists to decide that soaking MOFs in molecules that glow might be interesting.
But the real step would be constructing MOFs using these molecules as the links between the metal atoms. Doing this would solve one of the big problems with organic light-emitting molecules: they love to congregate. It turns out that these molecules are very much like people: give them a task and leave them by themselves, and the task will get done. But, tear down the walls, create an open office, and suddenly, you’re spending most of the time gossiping with your neighbors. Essentially, the molecules absorb the energy you give them, but instead of releasing it as light, they pass them back and forth among each other, losing energy as heat as they do so.
The only way to stop this is to keep the organic molecules separate from each other. And a MOF seems perfect for that. Essentially, if a light-emitting molecule was used to join the metal atoms to each other, then the light emitters are held in fixed positions that are relatively distant from each other. So MOFs should make the perfect substrate for organic light emitters.
But, they didn’t, or at least the experimental results were very disappointing. For example, a solution of light-emitting molecules under pretty good circumstances might emit four photons of light for every five photons absorbed. But the same molecule, used as part of a MOF, will only emit one photon for every four absorbed. With this sort of performance, it seemed that MOFs weren’t the answer.
Where does the energy go?
It turns out that the story is not over yet. As part of a MOF, the organic molecules are too far apart to pair up and lose the energy by passing it back and forth. But they’re not emitting much of it as photons. So where does the energy go?
It turns out that the absorbed energy travels around the MOF as an exciton. An exciton is an excited electron that pairs up with a positively charged area called a “hole.” The process of exciting the electron creates a hole where an electron should be, and that hole behaves just like a particle with the opposite charge to the electron. The electron and hole are held together as an exciton by their mutual (and eventually fatal) attraction.
The exciton travels around the MOF crystal for quite some distance. But the crystal is not that large, so, eventually, the exciton reaches the surface. Unfortunately, the surface is not exactly like the interior of the crystal. It isn’t just that the symmetry of the crystal is broken at the surfac but that the surface is not perfectly smooth. Some parts of the crystal grow a little faster than others, so you have steps, dips, and other imperfections. The exciton, traveling along this irregular surface, has plenty of opportunity to get trapped. Once in that trap, it will die without releasing any light. Instead, the crystal heats up a bit.
And that, it turns out, is a problem that can be solved. When they are on the surface, the excitons see all the metallic atoms at the corners that are sticking out by themselves—they miss the organic material in between (this is a drastic simplification).
Now, if you drop the MOFs in a plastic, then the plastic fills in for the missing links between the metal atoms. This smooths out the transition between the edge of the MOF and the rest of the world, while not providing the exciton a way to escape the MOF. As a result, more excitons end up choosing death by light emission, rather than death by heat.
In the end, the efficiency of light emission for MOFs encased in plastic almost returned to near perfect—seven photons emitted from ten photons absorbed, which is pretty good.
Why am I excited by this?
OLEDs and other light emitters are very good, and these MOFs have a long way to go before they can compete, but let me sketch out some of the possibilities for the future. One very cool possibility is color tuning through size selection. This is common in silicon nanocrystals and such-like, but it is not easily done using organic molecules. However, MOFs provide the advantages of both crystals and organics, so this becomes a possibility. That may not ever be useful in displays, but for scientific work, it is invaluable.
Then there is the issue of lifetime. As I said above, as organic molecules agglomerate, they stop emitting light. And, over time, this may be a problem with plastics that are infused with organic molecules. However, in MOFs, the molecules are held strictly in place by the crystalline order. That makes it more likely that they will last longer.
But, wait, there’s more! MOFs have huge empty spaces in them, so gases and fluids can quickly diffuse through their interior. These are just the right properties to make MOFs excellent detectors. It may be possible to use the presence of a MOF’s glow to sense low concentrations of target chemicals for safety or health diagnoses.
I’ve had to wait nearly a decade for this tiny amount of progress, but at least there is progress.
Nano Letters, 2017, DOI: 10.1021/acs.nanolett.7b04536
https://arstechnica.com/?p=1235323