There’s a pivotal scene in the 2012 film The Hobbit: An Unexpected Journey when Gandalf, Bilbo Baggins, and a company of dwarves are chased by orcs through a classic New Zealand landscape. For Northwestern University neuroscientist and engineer Malcolm MacIver, the scene is an excellent example of the kind of patchy landscape—dotted with trees, bushes, boxers, and rolling knolls—that may have shaped the evolution of higher intelligence in humans, compared to their aquatic ancestors. Specifically, it falls within a “Goldilocks zone”—not too sparse, and not too dense—that favors strategic thinking and planning ahead, leading to the development of “planning” circuitry in the human brain, according to MacIver’s most recent paper, published in Nature Communications.
This latest paper builds on earlier research. Back in 2017, MacIver and several colleagues published a paper advancing an unusual hypothesis: those ancient creatures who first crawled out of the water onto land may have done so because they figured out there was an “informational benefit” from seeing through air, as opposed to water. Eyes can see much farther in air, and that increased visual range could lead them to food sources near the shore. MacIver and his primary co-author, paleontologist Lars Schmitz of the Claremont Colleges, argued that this in turn drove the evolutionary selection of rudimentary limbs, enabling the first animals to move from the water onto land.
That hypothesis grew out of his research on the black ghost knifefish of South America, which is a nocturnal hunter that generates electrical currents in the water to sense its environment. After building a robotic version of the knifefish, with its own electrosensory system, he found that the volume of space in which it could detect prey (in this case, water fleas) was about the same as for a fish that relies on vision to hunt water fleas. The critical factor turned out to be that water absorbs and scatters light, limiting how far that light can travel: typically 10 centimeters to 2 meters, compared to the 25 to 100 kilometers light can travel in air.
Increasing eye size would have the effect of switching on high beams in foggy conditions so creatures could see farther, but eyes also require a great deal of energy to maintain, making such a development evolutionarily costly for ancient aquatic creatures. But larger eyes would confer an enormous evolutionary advantage in air, making the cost an acceptable tradeoff in a terrestrial environment, MacIver reasoned.
So MacIver and Schmitz scoured the fossil record for evidence of an increase in eye size during the critical water-to-land transition. They found that, over about 12 million years, there had been a tripling of eye size during that transitional period, from 13 millimeters before to 36 millimeters after. Even more intriguing, that increase began before the full transition from water to land was complete. This suggested that those aquatic creatures who learned to poke their eyes above water, like a crocodile, to find new food sources on the nearby shore had stumbled upon “the gateway drug to terrestriality,” MacIver told Quanta magazine in 2017.
The Goldilocks zone
Now MacIver is back with an even more provocative hypothesis: the geometry of certain habitats shapes evolutionary selection pressures in predator-prey contexts. “The basic idea is that open spaces—open grassland, flat plains—are just speed games, favoring the predator, since they are larger,” MacIver told Ars. “Closed spaces—dense forests or jungles—favor simple strategies of running for cover. Using a complexity measure, we show both of these habitats have low complexity.” That complexity measure is lacunarity, a geometric term for describing how patterns fill space.
The complexity “sweet spot,” according to MacIver, is a landscape like the one featured in The Hobbit chase scene, or like Botswana’a Okavango Delta, both of which feature an open grassland and moss zones dotted with clumps of trees and similar foliage. “In this zone, neither speed games nor running for cover maximizes survival rate,” said MacIver. “But planning—by which I mean imagining future paths and picking the best based on what you think your adversary will do—gives you a considerable advantage.” And that planning requires the kind of advanced neural circuitry typical of the human brain.
-
Botswana’s Okavango Delta has a patchy landscape that is a good example of the “Goldilocks landscape,” where the ability to plan results in a huge survival payoff.Tim Copeland
-
A chase scene in The Hobbit: An Unexpected Journey takes place over a New Zealand landscape that looks very much like the “Goldilocks zone.”
MacIver and his Northwestern colleague and co-author, Ugurcan Mugan, performed numerous supercomputer simulations, which revealed that while seeing farther (MacIver’s original theory) is needed for advance planning to emerge evolutionarily, it is not sufficient by itself. Rather, it requires a combination of long-range vision and complex landscapes. This, in turn, may have led to the development of one of the most difficult cognitive operations: envisioning the future.
MacIver and his team next built new predator/prey simulations to test survival rates of the prey under two different strategies. The first was habit-based, akin to entering a memorized password when prompted; the second was plan-based, involving the ability to imagine several scenarios and select the one with the best chance for survival. They used a simple landscape with no visual barriers to simulate a water environment and added various objects, with varying density distributions, to simulate land.
The results: in the simple water and land simulations, the prey showed low survival rates regardless of which strategy it employed, demonstrating that there was no evolutionary benefit to being able to plan in environments that are very open or too densely packed. In the former, the best bet is to try and outrun the predator; in the latter, there are too few clearly available paths, and the densely packed environment hinders how far the prey can see.
But a patchy landscape in the Goldilocks zone of complexity showed a huge increase in survival rates for prey that relied on the planning strategy, compared to the habit-based approach. MacIver’s team has developed an online game to illustrate how different landscapes (coral reef, jungle, savanna, and open water) affect our ability to plan and evade a stalking predator. (You can play it here.)
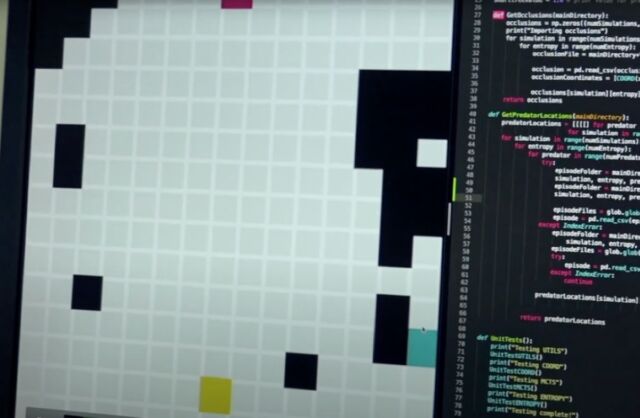
Now MacIver sees evidence of the Goldilocks zone everywhere, including movies like The Hobbit. “The use of space [in the chase scene] was just fascinating in terms of this interplay between opaque and open spaces,” MacIver said. While he hasn’t figured out how to measure the exact lacunarity of the landscape featured in that sequence, his hunch is that it would fall very close to his Goldilocks zone.
It likely was not a deliberate choice on the part of the filmmakers; MacIver sees it as an intuitive grasp of what makes for an exciting chase scene. “It’s about the anticipation of something that’s so powerful,” he said. “Seeing a scene unfolding at a distance and anticipating something bad, or good, is about to happen is what drives the drama.” A dense rainforest or similarly cluttered landscape would obscure the action, while a flat, barren plane would be too predictable. But the landscape featured in the film (and in the savanna) is just right. In those conditions, “Basically every movement becomes like a chess move,” said MacIver—and is thus far more entertaining to watch.
Not yet bulletproof
MacIver is the first to admit that more work is needed to “fully bulletproof” his hypothesis—particularly with regard to how it relates to the near-quadrupling in brain size that occurred in hominids after diverging from chimps. But his simulations “give some strong signal as to why it is that land animals ‘got smart’ while aquatic animals have not,” he said. (A possible exception is cephalopods, which do show signs of higher intelligence, perhaps because they were preyed upon by land animals, per MacIver.)
He is currently working with Northwestern’s Dan Dombeck on a follow-up study, testing his computational predictions via experiments in robotic environments. “We’ve created this complex space with repositional obstacles and controllable entropy, where there are two robots,” said MacIver. “One is a predatory robot driven by the same super-simple algorithm given in our paper. If it sees the prey, it runs toward it, for example. The other robot is the prey.” The prey has to get to the goal as the predator is in hot pursuit, just like in the online game.
More speculatively, MacIver thinks this could prove relevant to the question of why human beings struggle so much with thinking about looming existential threats, particular those in the distant future—climate change, for instance, or antibiotic resistant bacteria (or a global pandemic). “Inasmuch as you believe that the ability to think about the future was driven by a need to plan, and that our ability to think about the past is derivative of this need, a lot of who we are may hinge on why we evolved the circuitry to plan in the first place,” he said. “The reason we are so bad about planning for the distant future may redound to limitations in this circuitry that we have not yet developed the cultural technology to circumvent.”
DOI: Nature Communications, 2020. 10.1038/s41467-020-16102-1 (About DOIs).
Listing image by YouTube/Northwestern University
https://arstechnica.com/?p=1690430