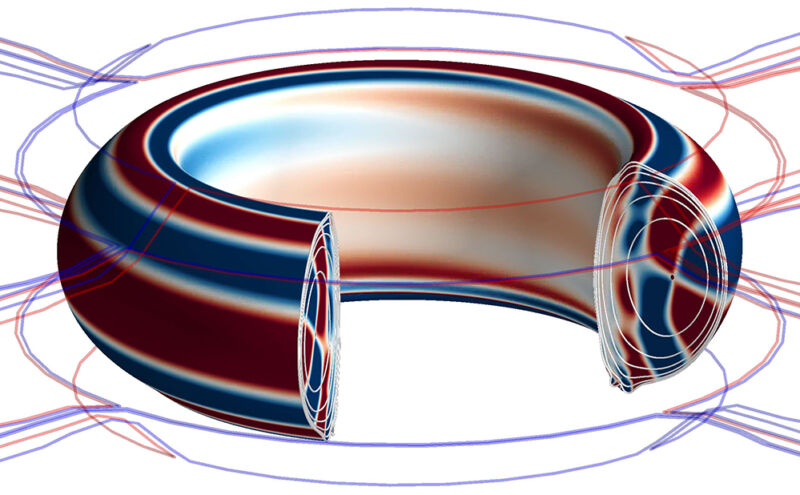
Depending on who you ask, fusion power is either already here (but no one will purchase my sekrit design!), never going to happen (so stop wasting money!), or a difficult problem that might be a partial solution to an even more difficult problem. The last, being the view of scientists who actually work in the field, is often lost in all the noise.
Out of this fog of discussion, a passel of papers emerged recently, all focused on a proposed fusion project: the SPARC tokamak. One of the surprising things about SPARC is its size. Coming in at just over 3m across, SPARC will be smaller than currently operating tokamaks, like JET, which is nearly 6m across. ITER, currently under construction in France, is over 12m across. Yet SPARC and ITER are projected to have about the same performance. Both are expected to produce more energy from fusion than the direct input energy, though neither is expected to produce useful power.
So why the difference? And what does this latest batch of papers tell us about the design?
Molten magnets and mad safety officers
In tokamaks, how you use it doesn’t matter; size matters. These devices confine a hot plasma using a donut-shaped magnetic field. One of these two needs to be big for the tokamak to work. If you can make a stronger magnetic field, you can get away with a smaller tokamak and still get the same amount of output power.
The magnetic fields are generated by coils that carry very large currents. Generating the current is not really a problem, but carrying it in a conductor is. If you use something like copper, then the power lost to the resistance of the wires will be huge, as power loss increases with the square of the current. Even worse, as the copper heats up, its resistance increases, leading to more heat generation, a shiny puddle of liquid copper, and a severely unimpressed safety officer.
Superconducting magnets are pretty much the only option. But all superconductors have a maximum current density. Exceed that and the conductor will start to show some resistance. Unfortunately, the materials from which superconductors are made are often poor normal conductors. Failing to obey the laws of physics here will lead an apoplectically angry safety officer and a bespectacled accountant trying to bill you for a large amount of helium and, possibly, a new building.
Once you know the maximum current you can carry, the magnetic field can be determined. From that, you choose how much power you wish to generate via fusion, and that sets the size of the tokamak. (Approximately; there are a few missing details in this process.)
Given this knowledge, and given that ITER was designed with state-of-the-art magnets, you’d have to conclude SPARC had trouble doing this math. Except ITER was designed in the 1990s and 2000s. Since then, high-temperature superconductors that have much better performance have been discovered and brought into commercial production. Using these modern superconductors, the magnetic-field strength can be doubled, allowing the size of the tokamak to be reduced considerably.
The future’s so bright?
While both ITER and SPARC are entering unknown territory, there is quite a bit of difference between the two projects. ITER has been modeled and studied to the nth degree. Teams of scientists have worked on every aspect for decades to try to predict the performance of ITER. SPARC, as a smaller device, cannot simply transfer the numbers and scale everything down by a factor of two. The recent papers try to address the challenge of modeling the new design.
They show that, fundamentally, SPARC seems sound. The plasma should reach the right conditions. The plasma should be able to maintain itself—it can carry a current that generates a magnetic field that helps confine itself—for about the same time as similarly sized tokamaks. That seems OK.
On the other hand, instabilities are likely to be exacerbated because the plasma is denser. In particular, phenomena called edge-localized modes could develop faster and be harder to suppress (or reduce). These instabilities occur at the edge of the plasma and, at worst, lead to hot plasma exhausting itself on the vessel walls. Other instabilities are disruptive in different ways, leading to reduced confinement and lower temperatures, so these generally need to be controlled.
These instabilities, if not controlled, can result in massive currents flowing in the vacuum vessel with extensive damage. This is the sort of scenario that gives ITER engineers nightmares, and the situation is not much different for SPARC: large currents, the whole machine jumping off its foundations, and other enjoyably dynamic disasters are possible. However, SPARC also seems to behave similarly to existing tokamaks, meaning that the predicted instabilities should be controllable.
A diverting puddle of tungsten
Where things really seem marginal is in the diverter. In every tokamak, there is a null point in the magnetic field. Particles don’t just leak through the null—they spray like a firehose. The diverter is the chosen place where this spray of particles hit a surface.
Even in current-generation tokamaks, the diverter materials don’t survive very long. In ITER, the diverter is going to be subject to conditions that are even more extreme. SPARC may make ITER look like warm milk.
Under their most pessimistic scenario, tungsten bricks will cyclically melt and recrystallize. During this process, tungsten atoms will probably penetrate to the core plasma, cooling it, and may even quench the fusion reaction. Carbon, an alternative, is a sacrificial surface that doesn’t kill the plasma. So carbon may end up being used in SPARC so that they can demonstrate that fusion works.
But the end result of using carbon will be organic molecules with a high percentage of tritium—not something to be messed around with. And definitely not something that should be considered for a commercial reactor.
Endgame
The success or failure of fusion rests on three pillars: science, engineering, and economics. ITER and SPARC will complete the science pillar. Assuming that we understand the physics pretty well—and I think we do—both tokamaks will show that fusion reactors that can produce useful electricity are possible. ITER will go a step further than SPARC and demonstrate many of the engineering requirements as well. Tokamaks will need to be cleaned robotically, for instance, and this is in the plan for ITER. The results from ITER can be transferred to future fusion reactors.
In any event, with sufficient work, the engineering case will probably be made. The economic case is much less certain. Most fusion researchers acknowledge this. The ITER-style large tokamak is a similar investment to the nuclear plants of the 1950s. And the payoff (if it ever arrives) is on a similar time scale. No one is sure who, if anyone, is prepared to risk that much money on that prospect. SPARC, by reducing the scale considerably, makes the economic case better by making the time scales shorter.
Weirdly, in retrospect, this makes the delays in ITER a lucky accident. Imagine if ITER had broken ground in 1990. By 2000, ITER would have been operating. The physics would have been worked out. The engineering path would have been clear. And the economics would have also been clear. In such an environment, the push for SPARC might never have come.
Journal of Plasma Physics, 2020, Special Issue
https://arstechnica.com/?p=1717051