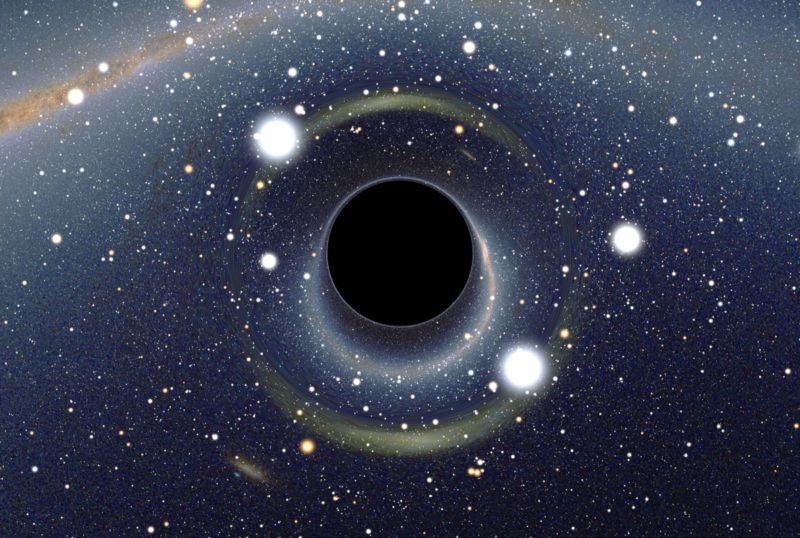
Israeli physicists think they have confirmed one of the late Stephen Hawking’s most famous predictions by creating the sonic equivalent of a black hole out of an exotic superfluid of ultra-cold atoms. Jeff Steinhauer and colleagues at the Israel Institute of Technology (Technion) described these intriguing experimental results in a new paper in Nature.
The standard description of a black hole is an object with such a strong gravitational force that light can’t even escape once it moves behind a point of no return known as the event horizon. But in the 1970s, Hawking demonstrated that—theoretically, at least—black holes should emit tiny amounts of radiation and gradually evaporate over time.
Blame the intricacies of quantum mechanics for this Hawking radiation. From a quantum perspective, the vacuum of space continually produces pairs of virtual particles (matter and antimatter) that pop into existence and just as quickly annihilate away. Hawking proposed that a virtual particle pair, if it popped up at the event horizon of a black hole, might have different fates: one might fall in, but the other could escape, making it seem as if the black hole were emitting radiation. The black hole would lose a bit of its mass in the process. The bigger the black hole, the longer it takes to evaporate. (Mini-black holes the size of a subatomic particle would wink out of existence almost instantaneously.)
Hawking’s prediction has enormous implications for theoretical physics, most notably for the black hole information paradox, but it has proven extremely difficult to test experimentally. The primary challenge is nicely summarized by Silke Weinfurtner, a physicist at the University of Nottingham, in a viewpoint that accompanies the latest results: “The temperature that is associated with Hawking radiation, known as the Hawking temperature, is inversely proportional to the mass of the black hole,” she writes. “And for the smallest observed black holes, which have a mass similar to that of the Sun, this temperature is about 60 nanokelvin. Hawking radiation therefore produces a tiny signal, and it would seem that the phenomenon cannot be verified through observation.”
So physicists have turned to so-called analogue black holes, first proposed in 1981 by University of British Columbia physicist William Unruh. He suggested a sonic analogue that he dubbed a “dumb hole,” since it is sound, not light, that becomes trapped behind a kind of event horizon. It’s a bit like a waterfall where the water flows faster and faster over an edge until it flows faster than the speed of sound through water. This creates the equivalent of a point of no return. The fluid is moving faster than the speed of sound, so no sound can outrun the fluid to escape in the opposite direction.
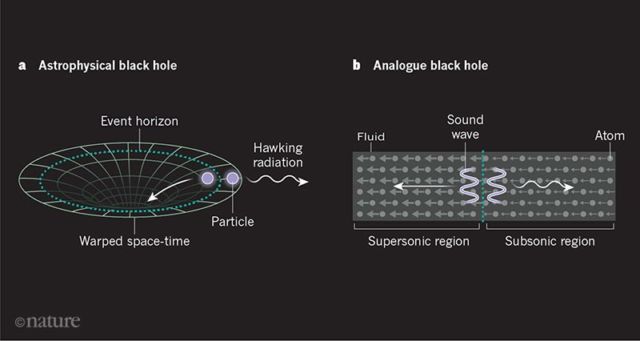
Unruh’s work was theoretical, but in 2009, Steinhauer briefly created a black hole analogue out of a Bose-Einstein condensate (BEC) made of 100,000 chilled rubidium atoms. When densely packed atoms are chilled to extremely low temperatures (billionths of degrees above absolute zero), they will collectively convert to their lowest energy state and essentially behave like one big “super atom.” That is a BEC, a kind of superfluity. Steinhauer was able to create the physical realization of Unruh’s dumb holes in a BEC—part of the fluid flowed faster than the speed of sound, effectively creating a supersonic region behind an event horizon that prevented sound waves from propagating in the opposite direction of the current.
Steinhauer reasoned that his analogue system should also emit the equivalent of Hawking radiation, complete with entangled phonons. In other words, sound waves should emerge from the sonic event horizon, just as Hawking radiation emerges from a real black hole. By 2014, he had observed tantalizing hints of the phenomenon, and two years later he announced the observation of entangled phonons being emitted by their sonic black hole. Granted, it wasn’t exactly Hawking radiation—it’s a sound wave analogue of it. But it was the strongest evidence to date in support of Hawking’s theoretical prediction.
Now Steinhauer is back with the results from his new, improved experimental setup capable of producing a much stronger signal. He and his co-authors have shown that pairs of sound waves—the sonic equivalents of virtual particles—emerge at the analogue event horizon. One is emitted away from the supersonic region while the other is absorbed. And just as Hawking predicted, “The system’s radiation… has a thermal spectrum with a temperature determined only by the system’s analogous equivalent to gravity, a relationship between the speed of sound and its flow,” Ryan Mandelbaum wrote at Gizmodo. “This means that it emitted a continuous spectrum of wavelengths, rather than preferred wavelengths.”
“The main novelty of de Nova and colleagues’ work is a clever detection scheme that they use to extract the temperature of the emitted radiation,” Weinfurtner writes. “The authors’ findings provide the first evidence of the Hawking temperature from a quantum simulator.” She notes further that this work might prove useful in measuring other unusual quantum phenomena likely to occur near the event horizon.
The next step is to repeat the experiment in hopes of measuring how this analogue version of Hawking radiation might change over time. “I’m interested in learning whatever we can about real black holes and real gravity,” Steinhauer told Gizmodo. “The way I see it, what we saw was that Hawking’s calculations were correct.”
DOI: Nature, 2019. 10.1038/s41586-019-1241-0 (About DOIs).
https://arstechnica.com/?p=1512087