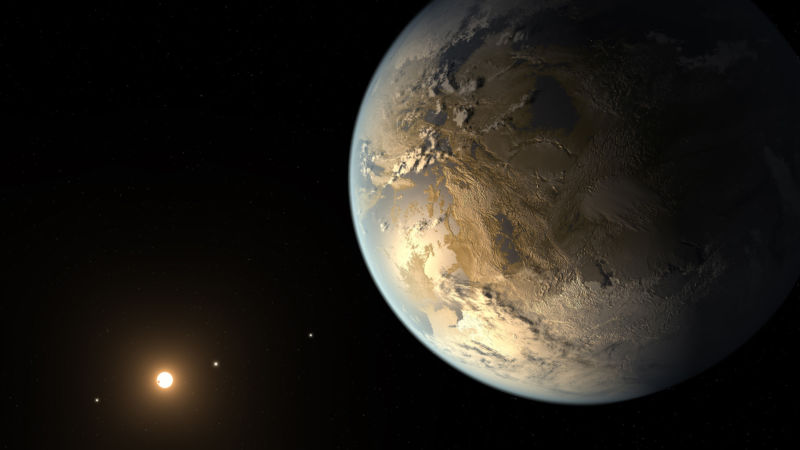
The last ten years will arguably be seen as the “decade of the exoplanet.” That might seem like an obvious thing to say, given that the discovery of the first exoplanet was honored with a Nobel Prize this year. But that discovery happened back in 1995—so what made the 2010s so pivotal?
One key event: 2009’s launch of the Kepler planet-hunting probe. Kepler spawned a completely new scientific discipline, one that has moved from basic discovery—there are exoplanets!—to inferring exoplanetary composition, figuring out exoplanetary atmosphere, and pondering what exoplanets might tell us about prospects for life outside our Solar System.
To get a sense of how this happened, we talked to someone who was in the field when the decade started: Andrew Szentgyorgyi, currently at the Harvard-Smithsonian Center for Astrophysics, where he’s the principal investigator on the Giant Magellan Telescope’s Large Earth Finder instrument. In addition to being famous for having taught your author his “intro to physics” course, Szentgyorgyi was working on a similar instrument when the first exoplanet was discovered.
Two ways to find a planet
The Nobel-winning discovery of 51 Pegasi b came via the “radial velocity” method, which relies on the fact that a planet exerts a gravitational influence on its host star, causing the star to accelerate slightly toward the planet. Unless the planet’s orbit is oriented so that it’s perpendicular to the line of sight between Earth and the star, some of that acceleration will draw the star either closer to or farther from Earth. This acceleration can be detected via a blue or red shift in the star’s light, respectively.
The surfaces of stars can expand and contract, which also produces red and blue shifts, but these won’t have the regularity of acceleration produced by an orbital body. But it explains why, back in the 1990s, people studying the surface changes in stars were already building the necessary hardware to study radial velocity.
“We had a group that was building instruments that I’ve worked with to study the pulsations of stars—astroseismology,” Szentgyorgyi told Ars, “but that turns out to be sort of the same instrumentation you would use” to discern exoplanets.
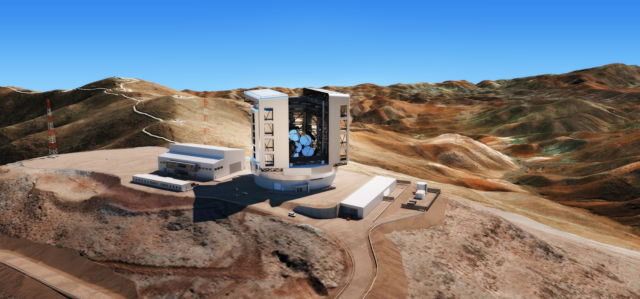
He called the discovery of 51 Pegasi b a “seismic event” and said that he and his collaborators began thinking about how to use their instruments “probably when I got the copy of Nature” that the discovery was published in. Because some researchers already had the right equipment, a steady if small flow of exoplanet announcements followed.
During this time, researchers developed an alternate way to find exoplanets, termed the “transit method.” The transit method requires a more limited geometry from an exoplanet’s orbit: the plane has to cause the exoplanet to pass through the line of sight between its host star and Earth. During these transits, the planet will eclipse a small fraction of light from the host star, causing a dip in its brightness. This doesn’t require the specialized equipment needed for radial velocity detections, but it does require a telescope that can detect small brightness differences despite the flicker caused by the light passing through our atmosphere.
By 2009, transit detections were adding regularly to the growing list of exoplanets.
The tsunami
In the first year it was launched, Kepler started finding new planets. Given time and a better understanding of how to use the instrument, the early years of the 2010s saw thousands of new planets cataloged. In 2009, Szentgyorgyi said, “it was still ‘you’re finding handfuls of exoplanetary systems.’ And then with the launch of Kepler, there’s this tsunami of results which has transformed the field.”
Suddenly, rather than dozens of exoplanets, we knew about thousands.
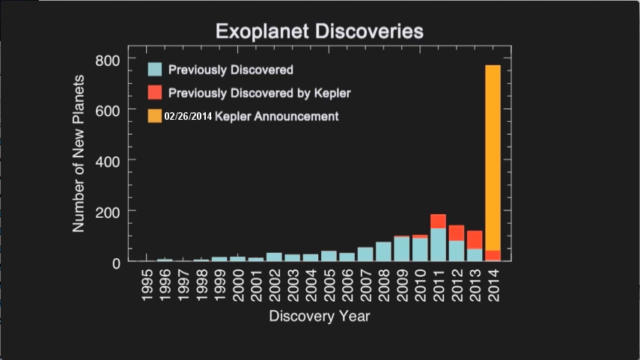
The sheer numbers involved had a profound effect on our understanding of planet formation. Rather than simply having a single example to test our models against—our own Solar System—we suddenly had many systems to examine (containing over 4,000 currently known exoplanets). These include objects that don’t exist in our Solar System, things like hot Jupiters, super-Earths, warm Neptunes, and more. “You found all these crazy things that, you know, don’t make any sense from the context of what we knew about the Solar System,” Szentgyorgyi told Ars.
It’s one thing to have models of planet formation that say some of these planets can form; it’s quite another to know that hundreds of them actually exist. And, in the case of hot Jupiters, it suggests that many exosolar systems are dynamic, shuffling planets to places where they can’t form and, in some cases, can’t survive indefinitely.
But Kepler gave us more than new exoplanets; it provided a different kind of data. Radial velocity measurements only tell you how much the star is moving, but that motion could be caused by a relatively small planet with an orbital plane aligned with the line of sight from Earth. Or it could be caused by a massive planet with an orbit that’s highly inclined from that line of sight. Physics dictates that, from our perspective, these will produce the same acceleration of the star. Kepler helped us sort out the differences.
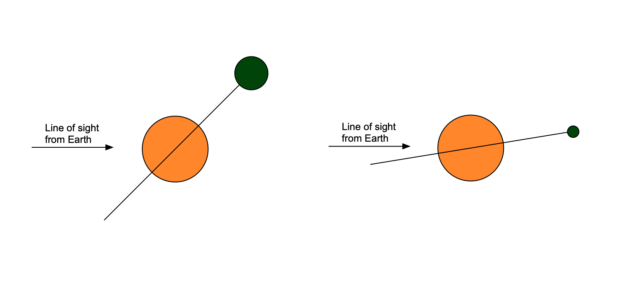
“Kepler not only found thousands and thousands of exoplanets, but it found them where we know the geometry,” Szentgyorgyi told Ars. “If you know the geometry—if you know the planet transits—you know your orbital inclination is in the plane you’re looking.” This allows follow-on observations using radial velocity to provide a more definitive mass of the exoplanet. Kepler also gave us the radius of each exoplanet.
“Once you know the mass and radius, you can infer the density,” Szentgyorgyi said. “There’s a remarkable amount of science you can do with that. It doesn’t seem like a lot, but it’s really huge.”
Density can tell us if a planet is rocky or watery—or whether it’s likely to have a large atmosphere or a small one. Sometimes, it can be tough to tell two possibilities apart; density consistent with a watery world could also be provided by a rocky core and a large atmosphere. But some combinations are either physically implausible or not consistent with planetary formation models, so knowing the density gives us good insight into the planetary type.
https://arstechnica.com/?p=1631693