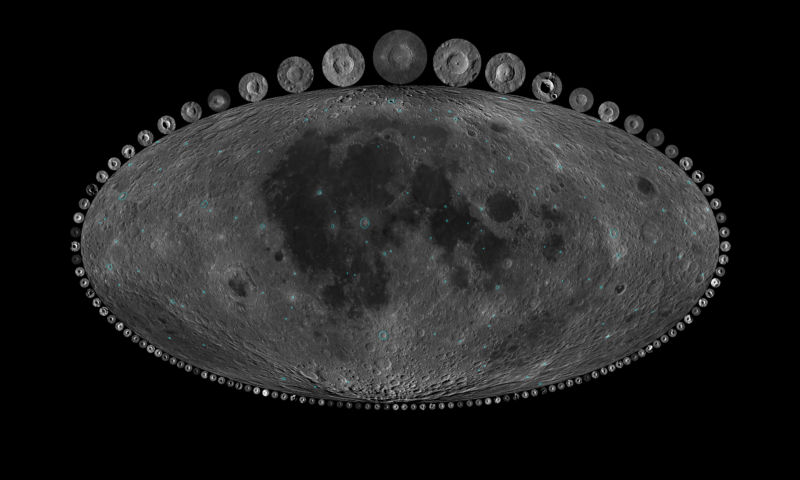
How often does a big rock drop on our planet from space? As we’ve gotten a better understanding of the impact that did-in the dinosaurs, that knowledge has compelled people to take a serious look at how we might detect and divert asteroids that pose a similar threat of planetary extinction. But something even a tenth of the size of the dinosaur-killer could cause catastrophic damage, as you could easily determine by placing a 15km circle over your favorite metropolitan center.
So, what’s the risk of having a collision of that nature? It’s actually hard to tell. The easiest way to tell is to look for past impact craters and try to figure out the frequency of these impacts, but the Earth has a habit of erasing evidence. So, instead, a group of scientists figured out a clever way of looking at the Moon, which should have a similar level of risk. They found that the rate of impacts went up about 300 million years ago.
Erasing history
Some impact craters on Earth are pretty obvious, but erosion and infilling with sediments make others much harder to find. We wouldn’t have noticed Chicxulub or the Chesapeake Bay Crater were there if we hadn’t stumbled across them for other reasons. As we go back in time, plate tectonics can erase evidence of impacts from the sea floor, as the rock they reside in gets subducted back into the mantle. And then, about 550 million years ago, the Great Unconformity wipes off any evidence of impacts that might have been left on land.
So, while we’ve come up with some rough estimates of impact rates on Earth, we don’t have a ton of confidence in them.
But there’s a nearby object without all the messy issues of plate tectonics, sediment deposits, and erosion. The Moon obviously preserves a clearer record of its impacts and is close enough that it should have a similar impact history to Earth’s. That’s in part because whatever it is that knocks an object out of a stable orbit and sends it toward Earth tends to break it up, creating a collection of fragments that gradually find their way to the Earth-Moon system. It may take millions of years for them all to hit, but the hits on both bodies should show a similar increased risk of impacts.
So how do you identify when impacts on the Moon happened? This is where the research gets very clever. The Moon’s surface is covered with a carpet of dust called regolith, formed as rocks are broken down by small impacts and charged particles. But a large enough impact will blast away the regolith and spray out chunks of solid rock, essentially resetting the process. Over time, this rock will gradually decay to regolith, and the extent of the decay should be proportional to the time since the impact.
Reading the heat
Unfortunately, we probably can’t convince China to send rovers to every crater to figure out how much regolith is there. So the researchers figured out how to do it remotely, using an instrument on the Lunar Reconnaissance Orbiter. It turns out that, during the lunar day, both rock and regolith get heated up by the Sun. Once the Sun sets, though, that heat starts to escape back out into space, where it can be detected as infrared radiation by the Lunar Reconnaissance Orbiter. But it escapes much more quickly from regolith than solid rock, meaning that, in the deep of lunar night, there’s still heat escaping from rocky features, while it has mostly gone from regolith-filled ones.
Starting with nine craters with known ages, the researchers confirmed that this is what we see on the Moon and calibrated the timing of the rocks’ decay. They then used this scale to estimate the ages of craters over 10 kilometers. This, incidentally, indicated that the thermal emissions of a crater dropped to the regolith background in about a billion years. So, while we can’t get a complete history of the impact rate, we can cover the Great Unconformity, as well as any recent changes.
One thing that was clear is that the size of things hitting the Moon hasn’t changed. The authors found that there was “no correlation between crater sizes and crater ages, meaning differently sized craters are randomly distributed in time.” But the rate of impacts did show a shift at about 400 million years ago, at which point the rate roughly doubled. In other words, we face a much higher risk (though still extremely low) of seeing an impact than the trilobites did.
Unfortunately, the uncertainty range was really large. The increase could be anywhere from 1.4x up to 20x, and its timing was similarly broad. So, the researchers turned to the Earth, performing a similar analysis using the age of craters 20km across and above. As expected, the Earth also saw a change in rate, and it was of similar magnitude to the one seen on the Moon. When the two rates are combined to a single measure, the uncertainties go down: the most likely date is about 290 million years ago, and the rate probably went up by 2.6x.
The authors suggest that the increase could be the product of one or more large asteroids in the main belt, which could send smaller bodies out that create a small “wave” of arrivals lasting hundreds of millions of years. If that’s the case, then there may only be a small population of bodies left in an orbit that puts Earth at risk of collision.
Again, the risks are small, and doubling a near-zero risk still leaves it at near zero. But the results make it clear that our Solar System is an active place even billions of years after its formation.
Science, 2019. DOI: 10.1126/science.aar4058 (About DOIs).
https://arstechnica.com/?p=1443231